PARTICLE PHYSICS
Nothing after Higgs
If the latest experiments at CERN don’t turn up something unexpected, an entire discipline is in danger of coming to a standstill. We take a look at the state of particle physics at a time when it’s under pressure.
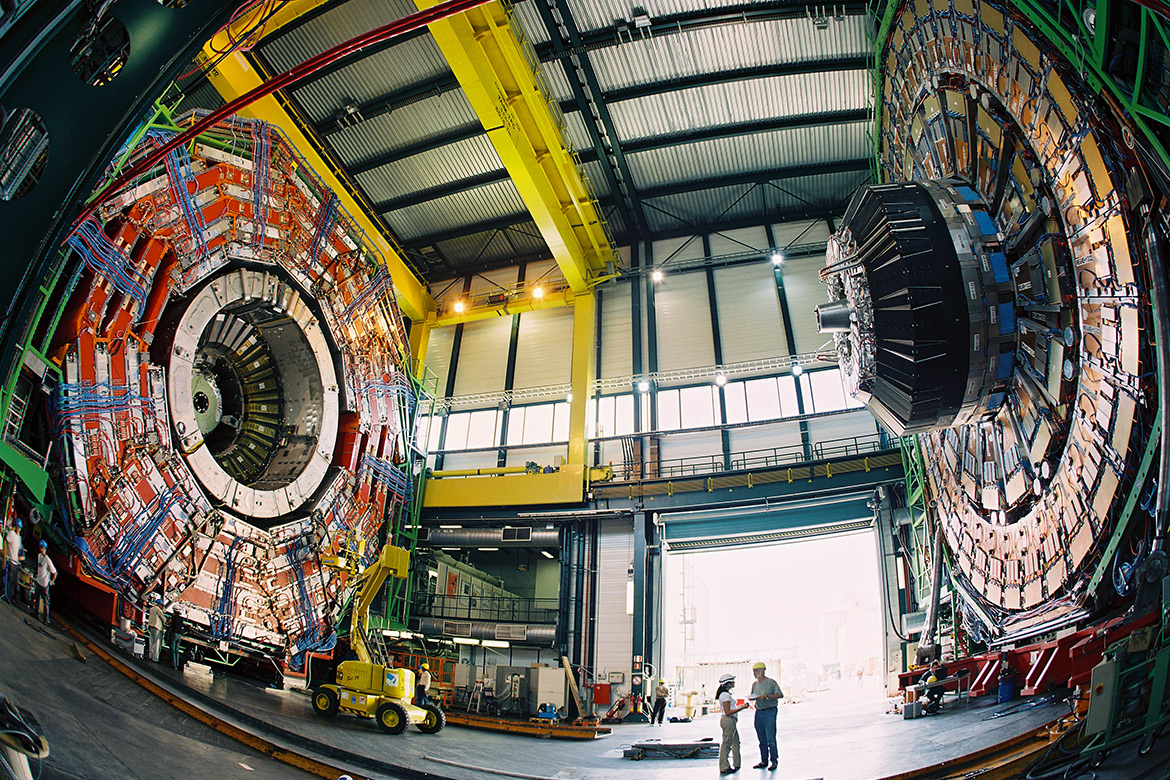
The CMS detector being assembled above ground in 2006. After being installed in CERN’s underground tunnel, it helped to detect the Higgs boson. | Image: CERN
On 4 July 2012, what was probably the biggest possible bout of euphoria among the 6,000 researchers working at the Large Hadron Collider (LHC) was seen at the CERN research centre. They had just detected the so-called Higgs boson. It confirmed a 45-year-old prediction: the last jigsaw piece in a theory called the Standard Model (see the box ‘The Standard Model: Beautiful but incomplete’). But not much has happened since.
A variety of exotic particles have been predicted by a host of theoretical physicists in recent years. But the hopes of finding them have not been fulfilled. “None of it has come to pass”, says Sabine Hossenfelder, a physicist at the Center for Mathematical Philosophy at LMU Munich. “It’s as if something has gone wrong at a fundamental level. This is how we’re going to lose whole generations of physicists”. The researchers at the LHC are now under pressure to find evidence of a new physics in their current measurement cycle.
After a three-year break at the LHC, protons are once again crashing into each other in its 27-km-long tube in order to create other particles and let the scientists observe their decay. They’d prefer to see particles that aren’t yet known. Indeed, they’re hoping that by focusing the particle beam better, and with a new energy record of 13.6 teraelectron volts (TeV), their disappointment will finally come to an end. “If they don’t find anything now, this field of research is dead”, the particle physicist Juan Collar of the University of Chicago told the journal Science in June 2022. This crisis is also bound up with the astonishing success of the Standard Model of particle physics. But researchers have long known that it can’t be an all-encompassing theory of everything, because it describes neither gravity nor dark matter. To this day, no one knows what this mysterious dark matter is made of, though it must make up the mass of 80 percent of the Universe. “A hundred years ago, Einstein and the Swiss physicist Fritz Zwicky were already pondering the problem that the fundamental theories of physics don’t fit together”, says Hossenfelder, who’s published a book entitled ‘Das hässliche Universum’ (The Ugly Universe). She’s critical of the LHC, complaining that there is a lack of fundamentally new ideas. “Good theories resolve a contradiction in existing theories”, she says. Approaches like string theory, which was once considered a candidate for the unified theory of physics, are interesting in principle. “But you still have to test them experimentally”, she says, “and particle accelerators are not suited for that”. Hossenfelder relies on testing theories in small laboratories. Many particle physicists – like Nicola Serra, a professor at the University of Zurich – have a less dramatic view of this apparent stagnation in particle physics. “We have reached a high level in our understanding”, he says. “So any further step is difficult. I think checkered progress is perfectly normal in such complex research fields. We have arrived at very profound questions that still need to be answered”. For example, neutrinos have a mass, but they’re treated as devoid of mass in the Standard Model. If so-called right-handed neutrinos were found, they could resolve this contradiction. They would also help to explain why there is as much matter as antimatter in the Universe, and they are also considered as possible candidates for dark matter itself. “Despite its significant successes, the Standard Model needs at least a minimal extension”, says Serra, who also conducts research at CERN. Another possibility for answering open questions is so-called supersymmetry – this is a mathematical extension that assigns a heavier partner to each known particle from the Standard Model. But none of these particles have been found. “Instead, the theories became ever more complicated”, says Hossenfelder. “And just because these mathematical extensions predict new particles doesn’t make it scientific. You’re merely producing an infinite number of papers that in my opinion are worthless”. Even without new particles, small discrepancies still keep appearing between the measurements of the Standard Model and its predictions. Serra is chasing down just such anomalies. The ‘Flavour puzzle’ is the name of the phenomenon he’s working on with his colleagues at CERN. It asks why the members of the families of matter particles have such a peculiar pattern in their mass – for example, why the light electron has two heavier partners, muon and tau. “Flavour anomalies need to be confirmed from the experimental and theoretical point of view, but if confirmed they may be a key component in solving this puzzle”, says Serra. However, a statistical correction means that the anomalies actually observed have meanwhile become smaller. The euphoria has dwindled with them. This is a recurring pattern in particle physics. Sensational reports are made, but after detailed examination, they’re often retracted again. This is what happened with the neutrinos that allegedly flew from CERN to the Gran Sasso Massif at faster than the speed of light in 2011. This result was caused by a defective cable and incorrect statistics – as the researchers realised in 2012. A similar tale might one day be told about the heavier-than-predicted W bosons at Fermilab near Chicago in 2022. At the time, many were talking about the discovery of a new physics. But the evaluations are still underway. “I don’t think they actually discovered anything”, says Hossenfelder dismissively. But Serra finds this laborious back-and-forth game perfectly normal between a possible new discovery and its subsequent correction. He himself has no intention yet of giving up his search for the Flavour anomalies. “We can still learn a lot at the LHC with the help of the new measurement data”, he says. “It’s a good thing to proceed so meticulously. But I think it’s unlikely that a new physics is going to come of it”, says Hossenfelder. “And this research needs a lot of money. You have to keep that in mind when people are calling for more powerful accelerators”. She’s referring to CERN’s plans to build a ‘Future Circular Collider’, a ring accelerator 100 km long and costing EUR 20 billion. She is by no means opposed to big science projects, preferring to keep an open mind about them. With the James Webb Space Telescope, for example, “we get our money’s worth, thanks to all the data from young galaxies that help us to gain a better understanding of dark matter”. But it’s also possible to test the fundamentals of physics more cheaply by using quantum optics or quantum computing, she says. More and more particle physicists are relying today on the possibilities of AI to search for unusual patterns of collision data. Steven Schramm, a physicist at the University of Geneva, hopes to use AI to find a new particle hidden in the noise of the LHC’s low-energy collisions (see Horizons 137, ‘Algorithms can fix it’). Nicola Serra is also counting on this technology to produce results: “AI could become a kind of co-pilot for particle physicists, seeing things that we humans can’t detect, maybe even suggesting new theoretical ideas”. Over the next 16 years, 16 times as much data than before is due to be collected at the LHC. Will this solve the torpidity of particle physics? Serra answers this with yet another question: “Should we rely on the potential of the LHC making a comprehensive array of measurements to harness the maximum information from it, and simultaneously develop new theories, or rather just wait for a new Einstein to suddenly pop up?” Dramatic stagnation – or the new normal?
Chasing down anomalies
Lots more data